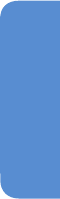
1023
Detection and Attribution of Observed Impacts Chapter 18
18
Dash, S. and T. Kjellström, 2011: Workplace heat stress in the context of rising
temperature in India. Current Science, 101(4), 496-503.
Davidson, E.A., A.C. de Araújo, P. Artaxo, J.K. Balch, I.F. Brown, M.M. Bustamante,
M.T. Coe, R.S. DeFries, M. Keller, and M. Longo, 2012: The Amazon basin in
transition. Nature, 481(7381), 321-328.
De Moel, H., G.M. Ganssen, F.J.C. Peeters, S.J.A. Jung, D. Kroon, G.J.A. Brummer, and
R.E. Zeebe, 2009: Planktic foraminiferal shell thinning in the Arabian Sea due
to anthropogenic ocean acidification? Biogeosciences, 6(9), 1917-1925.
De’ath, G., J.M. Lough, and K.E. Fabricius, 2009: Declining coral calcification on the
Great Barrier Reef. Science, 323(5910), 116-119.
De’ath, G., K. Fabricius, H. Sweatman, and M. Puotinen, 2012: The 27 year decline of
coral cover on the Great Barrier Reef and its causes. Proceedings of the National
Academy of Sciences of the United States of America, 109(44), 17995-17999.
Delaloye, R., C. Lambiel, and I. Gärtner-Roer, 2010: Overview of rock glacier
kinematics research in the Swiss Alps. Seasonal rhythm, interannual variations
and trends over several decades. Geographica Helvetica, 65(2), 135-145.
Dell, M., B.F. Jones, and B.A. Olken, 2009: Temperature and income: reconciling new
cross-sectional and panel estimates. American Economic Review, 99(2), 198-
204.
Dell, M., B.F. Jones, and B.A. Olken, 2012: Temperature shocks and economic
growth: evidence from the last half century. American Economic Journal:
Macroeconomics, 4(3), 66-95.
Delpla, I., A.V. Jung, E. Baures, M. Clement, and O. Thomas, 2009: Impacts of climate
change on surface water quality in relation to drinking water production.
Environment International, 35(8), 1225-1233.
Deschênes, O. and M. Greenstone, 2007: The economic impacts of climate change:
evidence from agricultural output and random fluctuations in weather. The
American Economic Review, 97(1), 354-385.
Deschênes, O. and M. Greenstone, 2011: Climate change, mortality, and adaptation:
evidence from annual fluctuations in weather in the US. American Economic
Journal: Applied Economics, 3(4), 152-185.
Descy, J.P. and H. Sarmento, 2008: Microorganisms of the East African Great Lakes
and their response to environmental changes. Freshwater Reviews, 1, 59-73.
Devictor, V., R. Julliard, D. Couvet, and F. Jiguet, 2008: Birds are tracking climate
warming, but not fast enough. Proceedings of the Royal Society B, 275, 2743-
2748.
Devictor, V., C. Van Swaay, T. Brereton, L. Brotons, D. Chamberlain, J. Heliola, S.
Herrando, R. Julliard, M. Kuussaari, A. Lindström, J. Reif, D.B. Roy, O. Schweiger,
J. Settele, C. Stefanescu, A. Van Strien, C. Van Turnhout, Z. Vermouzek, M. Wallis
De Vries, I. Wynhoff, and F. Jiguet, 2012: Differences in the climatic debts of
birds and butterflies at a continental scale. Nature Climate Change, 2(2), 121-
124.
deYoung, B. and A. Jarre, 2009: Regime shifts: methods of analysis. In: Encyclopedia
of Ocean Sciences [Steele, J.H., K.K. Turekian, and S.A. Thorpe (eds.)]. 2
nd
edn.,
Academic Press, Oxford, UK, pp. 717-721.
deYoung, B., M. Barange, G. Beaugrand, R. Harris, R.I. Perry, M. Scheffer, and F.
Werner, 2008: Regime shifts in marine ecosystems: detection, prediction and
management. Trends in Ecology & Evolution, 23(7), 402-409.
Di Baldassarre, G., A. Montanari, H. Lins, D. Koutsoyiannis, L. Brandimarte, and G.
Blöschl, 2010: Flood fatalities in Africa: from diagnosis to mitigation.
Geophysical Research Letters, 37(22), L22402, doi:10.1029/2010GL045467.
Diaz, R.J. and R. Rosenberg, 2008: Spreading dead zones and consequences for
marine ecosystems. Science, 321(5891), 926-929.
Diemberger, H., K. Hastrup, S. Schaffer, C.F. Kennel, D. Sneath, M. Bravo, H.F. Graf, J.
Hobbs, J. Davis, and M.L. Nodari, 2012: Communicating climate knowledge:
proxies, processes, politics. Commentaries. Current Anthropology, 53(2), 226-244.
Dietz, A.J., R. Ruben, and A. Verhagen (eds.), 2004: The Impact of Climate Change on
Drylands: With a Focus on West Africa. Environment & Policy, Vol. 39, Kluwer
Academic Publishers, Dordrecht, Netherlands, 465 pp.
Díez, I., N. Muguerza, A. Santolaria, U. Ganzedo, and J. Gorostiaga, 2012: Seaweed
assemblage changes in the eastern Cantabrian Sea and their potential
relationship to climate change. Estuarine, Coastal and Shelf Science, 99, 108-
120.
d’Orgeval, T. and J. Polcher, 2008: Impacts of precipitation events and land-use
changes on West African river discharges during the years 1951-2000. Climate
Dynamics, 31(2-3), 249-262.
Douglas, I., K. Alam, M.A. Maghenda, Y. Mcdonnell, L. McLean, and J. Campbell, 2008:
Unjust waters. Climate change, flooding and the urban poor in Africa.
Environment and Urbanization, 20(1), 187-205.
Doyle, M.E. and V.R. Barros, 2011: Attribution of the river flow growth in the Plata
basin. International Journal of Climatology, 31(15), 2234-2248.
Duarte, C.M., S. Agustí, P. Wassmann, J.M. Arrieta, M. Alcaraz, A. Coello, N. Marbà,
I.E. Hendriks, J. Holding, and I. García-Zarandona, 2012a: Tipping elements in
the Arctic marine ecosystem. AMBIO, 41(1), 44-55.
Duarte, C.M., T.M. Lenton, P. Wadhams, and P. Wassmann, 2012b: Abrupt climate
change in the Arctic. Nature Climate Change, 2(2), 60-62.
Duarte, C.M., I. Hendriks, T. Moore, Y. Olsen, A. Steckenbauer, L. Ramajo, J. Carstensen,
J. Trotter, and M. McCulloch, 2013: Is ocean acidification an open-ocean
syndrome? Understanding anthropogenic impacts on marine pH. Estuaries and
Coasts, 36(2), 221-236.
Dunne, J.P., R.J. Stouffer, and J.G. John, 2013: Reductions in labour capacity from heat
stress under climate warming. Nature Climate Change, 3, 563-566
Durance, I. and S.J. Ormerod, 2007: Climate change effects on upland stream
macroinvertebrates over a 25-year period. Global Change Biology, 13(5), 942-
957.
Durand, Y., G. Giraud, M. Laternser, P. Etchevers, L. Mérindol, and B. Lesaffre, 2009:
Reanalysis of 47 years of climate in the French Alps (1958-2005): climatology
and trends for snow cover. Journal of Applied Meteorology and Climatology,
48(12), 2487-2512.
Eakin, C.M., J.A. Morgan, S.F. Heron, T.B. Smith, G. Liu, L. Alvarez-Filip, B. Baca, E.
Bartels, C. Bastidas, C. Bouchon, M. Brandt, A.W. Bruckner, L. Bunkley-Williams,
A. Cameron, B.D. Causey, M. Chiappone, T.R.L. Christensen, M.J.C. Crabbe, O.
Day, E. de la Guardia, G. Diaz-Pulido, D. DiResta, D.L. Gil-Agudelo, D.S. Gilliam,
R.N. Ginsburg, S. Gore, H.M. Guzman, J.C. Hendee, E.A. Hernandez-Delgado, E.
Husain, C.F.G. Jeffrey, R.J. Jones, E. Jordan-Dahlgren, L.S. Kaufman, D.I. Kline,
P.A. Kramer, J.C. Lang, D. Lirman, J. Mallela, C. Manfrino, J. Marechal, K. Marks,
J. Mihaly, W.J. Miller, E.M. Mueller, E.M. Muller, C.A. Orozco Toro, H.A. Oxenford,
D. Ponce-Taylor, N. Quinn, K.B. Ritchie, S. Rodriguez, A. Rodriguez Ramirez, S.
Romano, J.F. Samhouri, J.A. Sanchez, G.P. Schmahl, B.V. Shank, W.J. Skirving,
S.C.C. Steiner, E. Villamizar, S.M. Walsh, C. Walter, E. Weil, E.H. Williams, K.W.
Roberson, and Y. Yusuf, 2010: Caribbean corals in crisis: record thermal stress,
bleaching, and mortality in 2005. PLoS ONE, 5(11), e13969, doi:10.1371/
journal.pone.0013969.
Ebinger, J.O. and W. Vegara, 2011: Climate Impacts on Energy Systems: Key Issues
for Energy Sector Adaptation. World Bank, Energy Sector Management Assistance
Program (ESMAP), The International Bank for Reconstruction and Development
/ The World Bank, Washington, DC, USA, 178 pp.
Eckert, N., C.J. Keylock, H. Castebrunet, A. Lavigne, and M. Naaim, 2013: Temporal
trends in avalanche activity in the French Alps and subregions: from occurrences
and runout altitudes to unsteady return periods. Journal of Glaciology, 59(213),
93-114.
Edwards, M. and A.J. Richardson, 2004: Impact of climate change on marine pelagic
phenology and trophic mismatch. Nature, 430(7002), 881-884.
Eijgelaar, E., C. Thaper, and P. Peeters, 2010: Antarctic cruise tourism: the paradoxes
of ambassadorship, ‘last chance tourism’ and greenhouse gas emissions.
Journal of Sustainable Tourism, 18(3), 337-354.
Eira, I.M.G., 2012: Muohttaga Jávohis Giella: Sámi Árbevirolaš Máhttu Muohttaga
Birra Dálkkádatrievdanáiggis (The Silent Language of Snow: Sámi Traditional
Knowledge of Snow in Times of Climate Change). Ph.D. Dissertation, University
of Tromsø, Tromsø, Norway.
Ekau, W., H. Auel, H.O. Pörtner, W. Gilbert, and D. Gilbert, 2010: Impacts of hypoxia
on the structure and processes in pelagic communities (zooplankton, macro-
invertebrates and fish). Biogeosciences, 7(5), 1669-1699.
Eliason, E.J., T.D. Clark, M.J. Hague, L.M. Hanson, Z.S. Gallagher, K.M. Jeffries, M.K.
Gale, D.A. Patterson, S.G. Hinch, and A.P. Farrell, 2011: Differences in thermal
tolerance among sockeye salmon populations. Science, 332(6025), 109-112.
Elliott, J.R. and J. Pais, 2006: Race, class, and Hurricane Katrina: social differences in
human responses to disaster. Social Science Research, 35(2), 295-321.
Elmendorf, S.C., G.H.R. Henry, R.D. Hollister, R.G. Björk, N. Boulanger-Lapointe, E.J.
Cooper, J.H.C. Cornelissen, T.A. Day, E. Dorrepaal, T.G. Elumeeva, M. Gill, W.A.
Gould, J. Harte, D.S. Hik, A. Hofgaard, D.R. Johnson, J.F. Johnstone, I.S. Jónsdóttir,
J.C. Jorgenson, K. Klanderud, J.A. Klein, S. Koh, G. Kudo, M. Lara, E. Lévesque,
B. Magnússon, J.L. May, J.A. Mercado-Díaz, A. Michelsen, U. Molau, I.H. Myers-
Smith, S.F. Oberbauer, V.G. Onipchenko, C. Rixen, N.M. Schmidt, G.R. Shaver,
M.J. Spasojevic, T.E. Thórhallsdóttir, A. Tolvanen, T. Troxler, C.E. Tweedie, S.
Villareal, C.H. Wahren, X. Walker, P.J. Webber, J.M. Welker, and S. Wipf, 2012:
Plot-scale evidence of tundra vegetation change and links to recent summer
warming. Nature Climate Change, 2(6), 453-457.